For an amateur rocketeer seeking to build a liquid rocket, one
of the most difficult components to obtain or build are remotely operated valves. A liquid rocket will require at
least one valve to start the flow of propellants to the combustion chamber. In the two small liquid rockets I
have flown in the last year or so, both used a pyrotechnic fire valve located between the pressurant tank and the
propellant tanks. The propellants were held in the tanks by burst disks (or equivalent) in the propellant run
lines. When the fire valve was actuated, the sudden pressure rise in the propellant tanks blew the burst disks,
allowing propellant to flow to the injector. This method of controlling the flow to the rocket allows the use of
only one valve, and eliminates liquid valves. In the case of the first rocket, the XLR-50 which flew in October
1993, elimination of the liquid valve was important because the oxidizer was liquid oxygen, and a small cryogenic
compatible valve is very difficult to construct. For the second rocket, which flew in October 1994, the small
size prevented the use of liquid valves. In fact, the single pryo valve I used was barely able to fit in the 1.5
inch rocket diameter. In this article I will describe the design of the valves that were used on these two
vehicles, and variations of them that have been used in other rocket applications.
Figure 1 shows the fire valve I used in the XLR-50 rocket. This valve supplied
helium to the propellant tanks, with an initial helium tank pressure of 800 psia. The design consists of an
aluminum piston with three O- rings used to seal between ports. In the pre- fire position, as shown in Figure 1a,
two of the O-rings seal the inlet , while both tanks are vented to atmosphere. To actuate the fire valve, a black
powder charge is ignited by application of 12 volts to a thin nichrome wire in contact with the charge. The rapid
expansion of the gases from the charge push the piston over against a stop, as shown in Figure 1b. In this
position, the helium tank is now connected to both propellant tanks, allowing them to quickly pressurize. The
tank vents are closed, with the fuel tank vent now allowing the excess squib charge gases to vent. Figure 2 Fire
valve for Micro Rocket The valve shown in Figure 1 consisted of a stainless steel body with a 0.375 inch diameter
piston. The O-rings were viton and the squib charge was contained in a Delrin plastic cap. The Delrin was used to
prevent shorting of the nichrome wire, and also to provide a frangible fuse in case the squib charge proved to be
a little too energetic. In practice I’ve never had the Delrin cap fracture. The inlet and outlet lines to the
tanks were silver brazed to the valve body. The valve was tested many times at inlet pressures of up to 1000 psi
without any problems, other than the O-rings would need replaced after several firings due to minor nicks from
the ports. To help alleviate this problem, the edges of the ports were rounded to help prevent the O-ring from
getting pinched as the piston translates. This was accomplished using a small strip of emery cloth that was
secured in a loop in one end of a short length of 0.020 stainless wire. The other end of the wire was clamped in
a pin vise which in turn was chucked in a hand drill. As the wire was rotated by the drill, the emery was pulled
snugly into the port, where it deformed into the shape of the inlet, and rounded the sharp edge. I used WD-40 as
a lubricant for this operation, allowing the emery to wear out until it would finally pull through the port. I
repeated this process a few times for each port until the piston would slide through the bore without the O-
rings snagging the ports. Another requirement is to lubricate the O-rings with a little Krytox grease. This helps
the piston move freely and greatly reduces the problem of nicked O-rings.
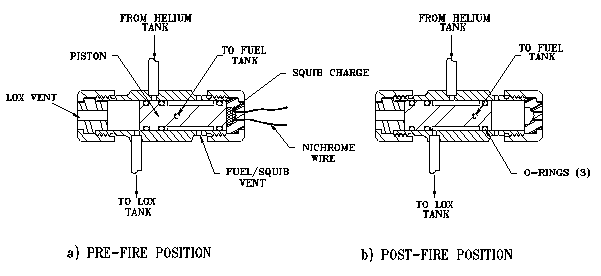
Fig 1. Fire valve for XLR-50 rocket.
The pyro valve I used in the 25 lbf thrust micro rocket that was launched in
October of 1994 is shown in Figure 2. This valve was identical in operation to the XLR-50 valve, with the major
difference being its integration into the vehicle body. The valve body was a 1.5 inch diameter aluminum bulkhead
that separated the nitrogen pressurant tank and the oxidizer tank. Because of the very small diameter of the
rocket, the clearances between ports and O- rings were minimized, just allowing the valve to fit. The fuel outlet
port was located at the vehicle center, providing pressure to the fuel tank by the central stand pipe that passed
axially down the oxidizer tank. The piston stop was a piece of heat treated alloy steel that was attached to the
valve body by a screw. This stop was originally aluminum, but was bent by the impact of the piston in initial
tests of the valve. The black powder charge in the Delrin cap was reduced and the black powder was changed from
FFFg to a courser FFg powder, but the problem persisted. The stop was re-made from oil hardening steel and the
problem was solved. In this application, the port diameters were only 1/16 inch so only a small amount of
rounding was required to prevent the O-rings from getting pinched in the ports. The valve operated with a
nitrogen lock-up pressure of 1000 psi.
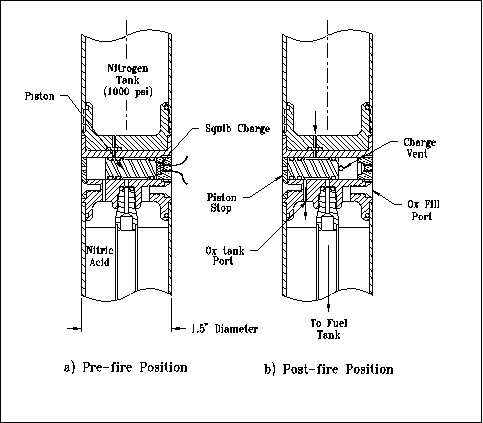
Fig 2. Fire valve for micro rocket.
A more challenging application of the same basic valve design was used for the
fire valve of Mark Ventura’s peroxide hybrid, as shown in Figure 3. This was the first application of this
valve where liquid was the fluid being controlled, rather than gas. In this case the liquid was 85% hydrogen
peroxide. The second difficulty was the fact that the ports were required to be 0.20 inch in diameter in order to
handle the required flow rate. The valve was somewhat simpler than the previous valves in that only a single
inlet and outlet were required. The valve body was made from a piece of 1.5 inch diameter 6061 aluminum, in which
a 1/2 inch piston bore was drilled. The piston was also 6061 with viton O-rings, which are peroxide compatible.
The ports were 1/4 inch pipe threads tapped into the aluminum body. The excess material on the sides of the valve
was milled off, so that the valve was only about 3/4 of an inch thick, and weighed only 4 ounces. Even though the
piston size was 1/2 inch, the same charge volume used in the 3/8 inch valves was sufficient to actuate the
piston. In testing the valve with water at a lock-up pressure of 800 psi, I was pleased to find that even with
the large ports, O-ring pinching was not a problem. One saving factor was that the larger size of the ports made
it easier to round the entrances on the bore side. The valve was tested with water several times successfully
before giving it to Mark for the static test of his hybrid. The only problem that occurred during the static test
of hybrid rocket was that the leads to the nichrome wire kept shorting against the valve body, Three attempts
were made before the squib was finally ignited and the engine ran beautifully. I have since been able to solve
this problem by soldering insulated 32 gauge copper wire to the nichrome wire leads inside the Delrin cap. In
this way I can provide long leads to the valve with reliable ignition.

Fig 3 Fire valve for Mark Ventura's hydrogen peroxide hybrid
rocket.
My next liquid rocket is a 650 lbf design that burns LOX and propane at 500 psia.
This engine uses a Condor ablative chamber obtained from a surplus yard. For this reason I call it the Condor
rocket. This rocket uses a scuba tank with 3000 psi helium for the pressurant. I decided to build a high pressure
version of my valve as the helium isolation valve for this rocket. When firing this rocket, just prior to the 10
second count, this valve will be fired, pressurizing the propellant tanks to 600 psi. I assumed going in to this
design that the O-rings slipping past a port simply wasn’t going to work at 3000 psi. At these pressures, the
O- ring would extrude into the port. In order to get around this problem I came up with the design shown in
Figure 4. For this valve, the O-ring groves were moved from the piston to the cylinder bore of the valve body, so
the O-rings do not move relative to the ports. The piston is made from stainless steel with a smooth surface
finish and generous radii on all of the corners. The clearance between the piston and the bore was kept very
small to prevent extrusion of the O-rings. The valve operation is similar to the one shown in Figure 3, and the
valve body is made in the same way except female AN ports were used rather than NPT ports. When the valve is
fired, the piston travels from the position shown in Figure 4a to that shown in 4b. During this travel, the inlet
pressure on the second O-ring will cause it to “blow out” as the piston major diameter translates past the
O-ring groove. The O-ring is retained around the piston, causing no obstruction or other problems. This valve has
been tested at 2400 psi inlet pressure with helium and works fine. It will be tested at 3000 psi prior to the
first hot fire tests of the condor rocket next spring. As a side note, essentially an identical valve design as
the one used on the Condor and Mark’s valve is shown in NASA SP- 8080, “Liquid Rocket Pressure Regulators,
Relief Valves, Check Valves, Burst Disks and Pyrotechnical Valves”.
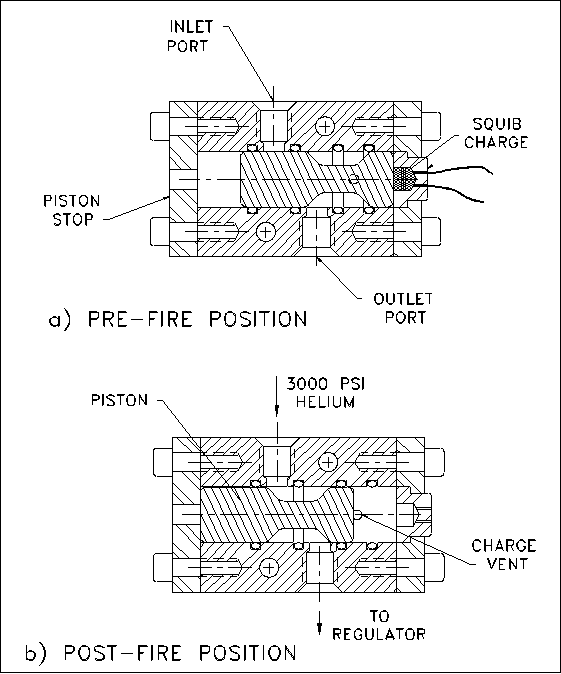
Fig 4. Condor rocket fire valve.
A second pyro valve is used on the condor system as shown in Figure 5. This
valve is used to vent the LOX tank in the event of a failure to open the fire valve to the engine. When the
propellant tanks are pressurized by the helium pyro valve, the LOX tank auto vent valve (shown in Figure 6)
closes. If the engine is not fired after a reasonable amount of time, the LOX will warm up, building pressure
until something gives (probably the LOX tank). The pyro valve shown in Figure 5 is used as the emergency tank
vent if the engine cannot be fired. The valve body is stainless steel with a stainless tube stub welded on for
connection to the LOX tank. This valve has been tested to 800 psi with helium and works fine. In this case, some
‘nicking’ of the O-rings can be tolerated because the O-rings are not required to seal after the valve is
fired. The ports in the bore are still rounded, however, to prevent the O-rings from getting nicked or pinched
during assembly of the valve. Figure 4 High pressure helium fire valve for Condor liquid rocket
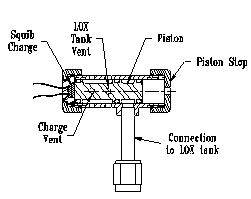
Fig 5. LOX tank emergency vent for Condor Rocket.
Even though it is not a pyro valve, I have shown the LOX auto-vent valve in
Figure 6 because this design has proven to be very useful for venting cryogenic propellant tanks without
requiring a separately actuated valve or control circuit. The valve uses a Teflon slider that is kept in the vent
position as shown in Figure 6a. This allows the tank to vent to the atmosphere, keeping the propellant at its
normal boiling point. When the helium system is activated, the pressurant pushes the slider closed against the
vent port, sealing off the LOX tank, as shown in Figure 6b. An O-ring is used around the slider to give it a
friction fit so the aspiration of the LOX tank does not “suck” the slider to the closed position. This
problem happened to David Crisalli when he scaled this design up for use on his 1000 lbf rocket system. I have
used this design on the LOX tank of my XLR-50 rocket, which used a 1/4 inch diameter slider, and on the condor
LOX tank, which uses a 1/2 inch slider. In both cases the vent valve worked perfectly. The main fire valve on the
Condor rocket is a pair of ball valves that are chained together to a single lever so that both the fuel and
oxidizer can be actuated simultaneously for smooth engine startup. For static testing of the rocket I will use a
double acting air cylinder to actuate the valves. For flight, however, I plan to use a pin that is removed by an
pyrotechnical squib to hold the valve in the closed position. When the squib is ignited, the pin is pulled by the
action of the charge on a piston, allowing the valves to be pulled to the open position by a spring. This method
may not be very elegant, but it is simple, light, and packages well on the vehicle. David Crisalli has
successfully employed this technique on his large rocket.
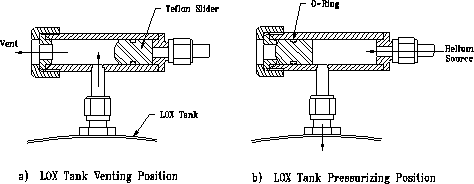
Fig 6. LOX auto-vent valve.
That covers the extent of the pyro valves I have built or plan to build so far.
In the next newsletter I will present the design and flight of the small hypergolic propellant Rocket that used
the valve shown in Figure 2. |